發展催化劑能在常溫常壓下有效能地轉換甲烷至甲醇
Develop a catalyst for catalytic conversion of methane into methanol efficiently under ambient conditions
The conversion of methane into methanol is very difficult chemistry requiring catalysts operating at elevated temperatures and pressures. The prospects of a future methanol economy depend on the successful development of robust and efficient catalysts that can accomplish this chemistry under mild conditions. In nature, the particulate methane monooxygenase (pMMO), a membrane-bound metalloenzyme, catalyzes methane oxidation with total selectivity and atom efficiency. Inspired by what we have learned about the pMMO over the past two decades, a tricopper complex [CuICuICuI(7-N-Etppz)]1+, where 7-N-Etppz stands for the organic ligand 3,30-(1,4-diazepane-1,4-diyl)bis[1-(4-ethylpiperazine-1-yl)propan-2-ol] based on the catalytic site of this enzyme has been developed and shown to mediate methane oxidation to methanol selectively. However, as a homogeneous catalyst, the catalytic efficiency and product yield are low because of the low solubility of methane in the solvent supporting the catalyst.
To overcome the problem of methane solubility in the catalytic system and to further improves the product yield. We re-formulated the tricopper cluster complex as a heterogeneous catalytic system assembled by immobilizing the tricopper cluster complex into mesoporous silica nanoparticles (MSNs) to take advantage of the “over solubility” of methane in liquids confined in nanoporous materials relative to the bulk solubility. For these gases, the interactions of the gas molecules with the nanoporous solid or with the solvent are significantly weaker than the interactions of the solvent molecules with the walls of the confining host framework. The stronger solvent–solid interactions create regions of low solvent density in the confined solvent, enhancing gas uptakes like CH4 and O2. The much higher solubility of methane within the pores of the mesoporous silica nanoparticles, as compared to the bulk solubility, led to very efficient turnover of the concentrated confined methane. Thus, the heterogeneous formulation exhibits dramatically higher catalytic efficiencies and turnover numbers, with commensurate improvements in chemical yields, offering the most proficient catalyst for the selective conversion of methane into methanol at room temperature developed to date. In this manner, we can drive the conversion of methane into methanol with hydrogen peroxide with a catalytic efficiency approaching 100%, as well as with high energy and atom economy under ambient methane pressures. The heterogeneous catalyst is robust and reuseable at least three reaction cycles. The samples are easily separated from the reaction mixture and dried under vacuum after each reaction cycle before performing the next run. This technology will lead to an economic process for methanol production that could have potential for further commercial applications.
直接轉化甲烷至甲醇是十分困難的化學反應,通常需要操作於高溫高壓的條件下。而在未來甲醇經濟(是一種提議中未來的經濟形式,使用甲醇來代替現在廣泛使用的化石燃料來用作能量存儲,地面交通燃料,以及合成碳氫化合物的原料及其產品)的前景取決於是否能研發出強壯以及高效率的催化劑,能於常溫常壓下轉化甲烷為甲醇。然而在大自然中,普遍存在於嗜甲烷菌中的細胞質內膜上的微粒體型甲烷單氧化酵素(pMMO)卻可在常溫下高選擇性轉換甲烷為甲醇。從過去二十年研究微粒體型甲烷單氧化酵素所學的知識並模擬其酵素活性中心的結構所合成出的三銅金屬錯合物[CuICuICuI(7-N-Etppz)]1+,可以於常溫常壓下高選擇性地執行甲烷至甲醇的反應。然而這種昀相催化劑的催化效率以及甲醇的產率仍然十分低,最主要的原因是甲烷在有機溶劑中的溶解度十分低所致。
為了改善甲烷氣體在催化系統中的溶解度,以近一步提高甲醇的產率,我們將三銅金屬錯合物負載於官能基化的中孔洞二氧化矽奈米球材料的垂直孔道中,利用”過度溶解度”的特性來大幅度提升被限制於奈米孔洞中的甲烷的溶解度。在限制空間中,由於非極性的甲烷氣體分子與奈米孔洞內壁以及溶劑之間的作用力顯著的弱於溶劑分子與奈米孔洞內壁骨架間的作用力,而這溶劑與骨架間的強作用力造成許多區域的溶劑分子濃度較低但非極性氣體分子的濃度大幅提高(例如氧氣及甲烷)。高濃度的甲烷分子貯存於二氧化矽奈米球的孔洞中,可以顯著的提升甲烷的催化效率以及轉換數,有效率的提升產物甲醇的產率,並有可能發展成為至今最有效率的高選擇性常溫常壓下轉換甲烷為甲醇的催化劑。此異相催化劑甚至可以達到百分之百轉換甲烷為甲醇,有很高的能量與原子的經濟性。此外此催化劑也可以重複使用至少三次催化反應。此項技術, 將邁向更經濟的直接甲烷氧化為甲醇的工業製程並十分有潛力於商業應用。
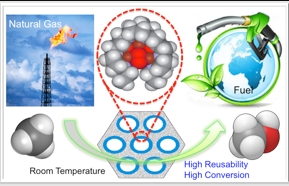